Perhaps you've heard the sentiment that the first trillionaire will be an asteroid miner. Or perhaps you read too much scifi as a kid (or like me, still do). Or perhaps you've signed on to the abundance hype of the 4th industrial revolution (or is it the 5th now?). Regardless, you've set your sights on 16 Psyche, pickaxe in hand.
Not so fast, wrangler...
I admire your moxie, but unfortunately we're gonna hafta burst a few bubbles here: We don't currently have the capability.
"WHAT?" you say.
We don't even have the capability to recycle satellites in Low earth Orbit (LEO).
"Who's this 'we', Padre, and what does one have to do with the other?"
We as in human civilization in 2023. As far as how asteroid mining and satellite recycling are related, let's start with the logistics of orbital In Situ resource utilization (ISRU). Whether it's an asteroid or a satellite, you have to do the 7 steps shown to the right. The order might change depending on your approach, but they all need to be considered.
So...
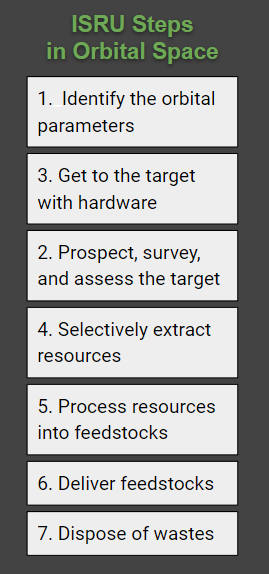
1. Identifying orbital parameters tells you where the target is and where it will be at any given time and allows you to plan a mission. For satellites around Earth, we have very good tracking for anything larger than 10cm. For asteroids, the smallest one we know of is 2015 TC25, which is about 2m across. Obviously, knowing parameters is easier the closer stuff is to Earth. Orbital parameters include things like semimajor axis diameter (a), plane/inclination (i), eccentricity (e) that determine mass and transportation costs (i.e., "delta-V"). They also include parameters such as longitude, periapsis, and epoch that dictate launch and burn timing. We won't worry too much about those as we will assume commercial interests will be looking to time optimally for minimum expenditure.
2. Prospect, survey, and assess the target for a satellite is as easy as calling up the satellite owner and getting them to send you a bill of materials (BOM), a materials and processes list (MPL), and any CAD data or blueprints (for older sats). It's all on file somewhere. For an asteroid, it's not so easy. There are basically three major categories of asteroids, mostly C type (mostly made out of carbonaceous clay possibly with some water), some S type (silicates), and the heavier M types (metals). You can sort of generally tell what kind of an asteroid it is by its albedo (how reflective it is), but to know exactly, you have to send equipment there, which leads us to...
3. Getting around in space means delta-V. Every orbit around the Sun (or around Earth, for LEO) has an associated velocity. To go from one orbit to another means a change in velocity, or delta-V. The rocket equation converts delta-V to cost, measured in propellant mass. Just changing the size of an orbit is a pretty simple calculation; changing the plane (inclination) is more complicated and much more expensive, in delta-V. So let's compare delta-V for near Earth resources. The green bars are derived from the Union of Concerned Scientists satellite database, and show the number of satellites for each delta-V (bucketized into 0.1km/s increments) relative to a notional depot. Since this fictional depot doesn't exist yet, we have the freedom to put the depot where it will make the most business sense, which is where the most mass is in LEO, at the ISS (for more on this please visit the RecycleISS petition). The yellow histogram shows a similar chart pulled from the JPL calculations for Near Earth (NEO) Asteroids, that shows the delta-V costs for all the known asteroids in vicinity of Earth's orbit. The cheapest resources are close to 0 km/s delta-V (the ISS orbit at ~51 deg inclination, 450km altitude). The cheapest resources for asteroids are over 4 km/s from Earth transfer orbit, which is where it makes sense to put a refining depot (more discussion on why in section 6). Also, what these charts show is the minimum delta-V; if you are impatient and want your stuff to arrive faster, or launch outside of nominal windows, it will cost more delta-V.
"But," you say, "the mass of asteroids out there is astronomical compared to those dinky satellites!"
You have to consider that lots of asteroids at the same delta-v does not mean they are at the same location, direction or even in the same plane. Kinda like Boston and Fresno are both 1,600 miles from Wichita- which it oughta make sense then why we look at the number of objects rather than the mass for the first pass, because each target is its own mission. Resource mass just lets you prioritize missions (and we may look at that in the future, but the mass of asteroids in the various databases are still mostly blank). For now, note that the delta-V is each way and doesn't account for the mass you are moving. The actual propellant cost depends on your propellant source and the amount of mass you are moving, dictated in general by the rocket equation. To compare apples-to-apples though, we need to keep things on a per kg basis. If you are going to propose ISRU propellant manufacturing or solar sails as the solution for one set, you need to apply it to the other set. More on this in step 6. Also, the stuff in LEO is growing exponentially, doubling about every two years, whereas the asteroids are pretty much the same ones that have been there for millions of years.
4. Resource extraction, or "mining" in space brings a whole set of challenges. Just on Earth, mining technology is its own specialty combining elements of civil, mechanical, & chemical engineering with materials science and geology, and it all has to be reinvented for microgravity. Rendevous/capture is the first thing that has to happen. You don't really "land" on an asteroid any more than you "land" on a satellite. Bouncing off is more the reality, unless you have a way to attach. Attaching to a satellite can be tricky if it's spinning, but at least there's usually something to grab onto. Once you've done that, you can move the whole thing around (at the cost of delta-v/propellant). Asteroids, on the other hand, can also be spinning, and most of them are what's known as "pile-of-rubble" bodies, essentially gravel and electrostatic dust. To "grab" an asteroid, you pretty much have to grab the whole thing in a way that it doesn't break apart. There are people working on this challenge, but it's a lot farther from realization than a simple robotic gripper. Once it's capured and contained, it needs to be taken apart.
5. Selectively extracting different materials is needed because different materials have to be processed differently. And, like every other technology we've developed in the last 10 thousand years, they are all currently based on 1-g gravity. Overcoming the challenge of translating processes for microgravity is where Lunexus excels, but there are a LOT of processes that have to be redesigned for microgravity and very few have yet been demonstrated by anyone yet. For now, let's compare based on physics and what's being processed. For a satellite, every material contained in it is already a space grade material (metal alloys, polymers, and silicon). By contrast, asteroids depending on type, are typically comprised of various impure ores. Ores are metal oxides, and the refining process of turning ore into raw metal is known as smelting. It's an energy intensive process with lots of waste products. For aluminum (a common structural material for spacecraft with relatively low temperatures) the smelting energy is 15 kWh/kg. This step is typically not needed for satellites, which as mentioned are mostly (by mass) already refined space grade alloys.
Once you've smelted those various ores (for the case of asteroids), you need to store the raw metals and when you've accumulated enough, then you need to mix them into the desired alloys, and form into the feedstock of choice (e.g., wire, extrusions, billets, etc.). This is a process shared with satellite metals recycling, and requires metals to be remelted. They are mostly the preferred alloys already, but that may change as space materials technology progresses, so it will be good to develop alloy mixing regardless. Melting refined aluminum requires about 1 kWh/kg.
In the case of aluminum, then, it requires about 16X less energy to process a kg of alloy feedstock from a satellite than it does to get it from an asteroid. While the temperatures and specific energy required will vary by material (other metals such as steel and titanium are much higher in energy, and the processing for polymers and silicon may require somewhat different approaches), the energy factor between processing raw ores and recycled space grade materials will generally be greater than 10X in favor of satellite resources.
6. Delivery of feedstock to customers is the fun part because it's the part where you get paid for all the work you did to get to this point. Who your customers are sort of dictates where they are at, but that is at least partly dictated by where their customers are at. Currently all end customers are on Earth. That will not change until infrastructure is built up in other locations like the Moon, or L2, or Mars and people with needs and money live off Earth. Manufacturing anywhere off Earth will make sense when the product sales price/kg is substantially higher than the cost/kg to get the feedstock delivered, and the production technology actually exists. Below are a few case studies in potential markets for space resources, that perhaps illustrate why space is such a hot area right now.
Info Sat Industry
Comsats, GPS, Earth Observation
$20B Market
-
CAGR 15-18%
- Feedstock Cost: $0/kg
- Revenue/kg: $∞
ZBLAN Industry
Advanced Matl Fiber Optics
$5-7B Market
-
CAGR ?%
-
Feedstock Cost: $3,500/kg
-
Revenue/kg: $10,000
Biopharm Industry
3DP organs, synthetic proteins
$6-8B Market
-
CAGR ?%
-
Feedstock Cost: $3,500/kg
-
Revenue/kg: $10,000
OSAM Sat Industry
Satellite component mfg on orbit
$20B Market
-
CAGR ?%
-
Feedstock Cost: <$3,500kg
-
Revenue/kg: $10,000
The only one of these cases that currently exists is the commercial sat industry, which sends up sats and sends back data (zero mass). The middle two cases are very similar and illustrate actual physical products sold at high prices which supports the model of manufacturing in space. These materials leverage microgravity production, but feedstocks are still incredibly expensive to deliver from Earth, and then return back down at upwards of $10k/kg round trip. The OSAM (Orbital Services Assembly and Manufacturing) case illustrates an often neglected but exisitng market (the commercial sat one we already discussed) that can be tapped by manufacturing components for it on orbit. We think this is the biggest opportunity in space right now, if it can source material economically in space. The customer is in LEO, just need to locate the depots close by.
7. Disposal of wastes is rarely considered on a first pass of terrestrial economics, and a greenhorn asteroid miner might be inclined to just neglect this part altogether. However, for a lot of good reasons, at Lunexus, that's not the way we roll. One of those reasons is that the rocket equation tells us just how valuable mass in space is. With the right technology, any mass can be used as a propellant. Another reason is, as we are seeing in LEO now, mass left unattended can get in the way of future hardware and spacecraft transits. "In the way" is kind of like being in the way of a bullet (v = 1 km/s), except it's usually going to be larger and more pieces, like if a cannon and a shotgun had a baby and it took steroids to go faster (upwards of 30km/s). It's totally not cool to leave it flying around like that. Anything we process in space needs to be bagged and tagged with a beacon, even in deep space. It's ok to vaporize matter if you are using for propellant. Otherwise leave it intact for future wranglers to use.
So Pardner, that's the lowdown on space logistics. Along the way we touched on some of technological challenges of reinventing every industrial process we need for microgravity environment.
You ask, "What do we need to close the technology gap?"
The simple answer is: a good reason to develop that tech. One approach is to recycle the ISS. We've collaborated with others in the industry to create this petition and bring attention to the travesty of vaporizing the space station in 2030. On Fig 1 that you saw previously, it represents the single largest concentration of resources in LEO and easiest concentration to access in near Earth orbital space. Let's use it to build and test processing technology, create a circular economy and be ready to wrangle those asteroids!